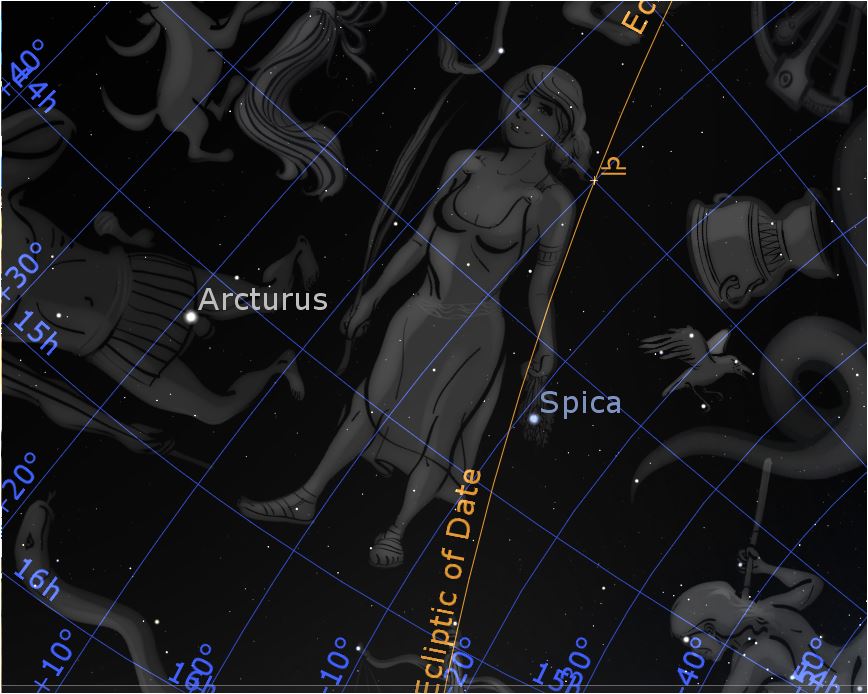
The most suitable stars for measuring the coordinates of the precession of the AEQ/VEQ points are those that are visible near the Ecliptic.
Spica is next to the Ecliptic, in the constellation of Virgo.
(In the middle of the goblet held in the left hand of the virgin sign, as shown in the photo above.)
Spica is easy to observe today on AEQ days because it appeared above the horizon when the Sun dipped below, and the sky darkened. This was not the case in ancient times. It is known that the coordinates of Spica in antiquity were measured primarily indirectly through the coordinates of the moon. The reason for this is as follows:
In the centuries of Timocharis, Hipparchus and Ptolemy, the celestial point of the autumn equinox, the AEQ point, was virtually very close to Spica. Today, the AEQ point is further away from Spica, as the photo above shows. Around the AEQ day, the Sun is also close to the AEQ point in the sky. This means that Spica also appears close to the Sun around the date of the AEQ. Therefore, in the centuries above, around the AEQ day, Spica was not visible to the naked eye because the Sun blinded the observer, as shown in the figure below.
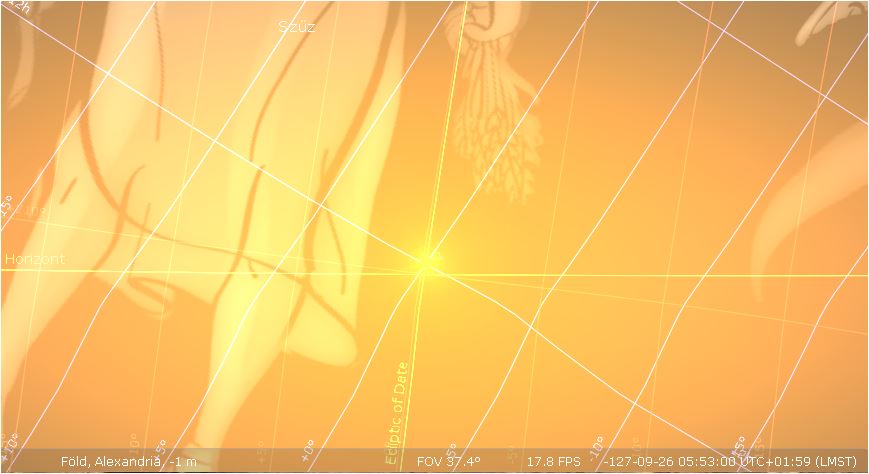
Spica went under the horizon just before sunset and rose above the horizon just before sunrise. The following photo is darkened to show Spica just above the green line of the horizon and the Sun just on the horizon. (Of course, you can’t look below the horizon, only Stellarium allows you to do that.)
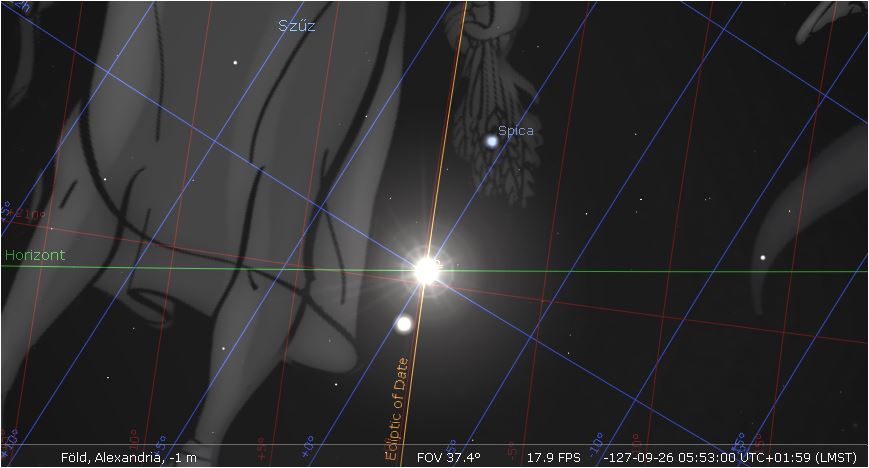
Both photos above were shot by the blogger with Stellarium at Sunrise on AEQ day of 128BC, in Alexandria, Egypt.
Fortunately, the full moon is just opposite the Sun. So, the coordinates of the Sun can be determined easily by measuring the coordinates of the moon. This was a usual procedure in antique astronomy.
Consequently, the simultaneity of the AEQ day and the full moon day could play an essential role in measuring the celestial position of the Spica. Note: Of course, the relative coordinates of Spica and the other stationary observable stars near the ecliptic plane (Alpha LEO; Alpha CMi, etc.) also supported the determination of the coordinates of Spica.
Let us look at the years of measurement from this point of view.
It can be observed that in two of the measurement years mentioned (278BC (-279); 128BC (-127)), AEQ and the full moon coincided. But the currently hypothetical year AD138 was not such an AEQ & full moon year.
It looks likely that all three years of measurement had to be coincident AEQ & full moon years.
However, instead of the uncertain AD138, the year AD131 would have been more appropriate, as this is the closest AEQ & full moon year.
AD150 could also fit but is out of the question because it is already the year of publication of Ptolemy’s very long and earlier written work.
The photo below was taken on 24 September AD131, on the day of the AEQ, about 1 hour before sunrise, and shows the sky from Alexandria. The Sun is still below the horizon, marked by the horizontal green line, near the AEQ point, the intersection of the Ecliptic and the celestial equator. Spica is to see in the circular frame next to both the AEQ point and the Sun. The coordinates of Spica are shown in the list on the left of the photo. It can also be seen that Spica is just above the Sun, and it will rise slightly before the Sun but will not be directly observable due to the Sun’s blinding proximity. (Stellarium photo by the blogger.)
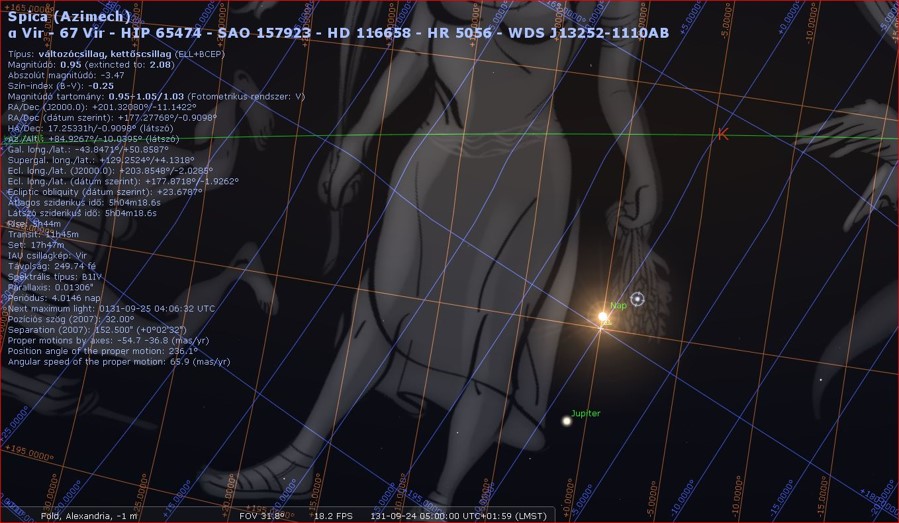
The following photo is a wide-angle view of the East-West sky on AD131 on 24 September, on the day of AEQ, at the same time as the photo above. The full moon is well visible above the horizon, near the symbol for the VEQ celestial point, the other intersection of the Ecliptic and the celestial equator. The full moon is positioned opposite the Sun but will soon dip below the horizon. The coordinates of Spica can be calculated indirectly from the coordinates of the full moon. (Stellarium photo by the author.)

Let us have a look at the Ecl. long. coordinates of Spica on the dates of measurings as we see it, on the possible dates of AEQ and full Moon coincidence:
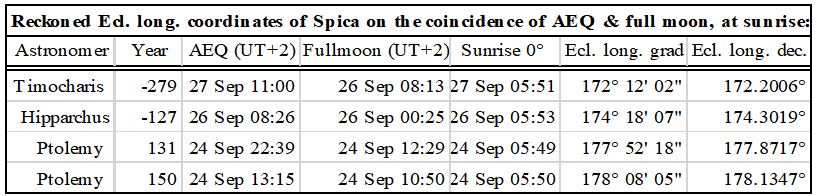
Table 4.
The above Table 4. is based on the data of Stellarium. It shows the changing of the ecliptical longitudinal (Ecl. long.) coordinates of Spica. The ecliptical longitudinal coordinates are traditionally measured from the VEQ point’s longitude zero. That is why the coordinates of Spica (and not those of the AEQ point) are changing.
Table 4. tells us, too, that the Ecl. long. of Spica gradually “approached” the AEQ point, which is at 180° Ecl. long. This table plays an essential role in the following.